The Natural Combination Medicine Traumeel (Tr14) Improves Resolution of Inflammation by Promoting the Biosynthesis of Specialized Pro-Resolving Mediators
Abstract
The resolution of inflammation is an integral part of the acute inflammatory response and eventually leads to the return to homeostasis. It is supported by specialized pro-resolving mediators (SPMs) that act as immunoresolvents via specific G-protein-coupled receptors. In contrast to classical non-steroidal anti-inflammatory drugs (NSAIDs) that suppress the formation of pro-inflammatory lipid mediators such as prostaglandins, novel pharmacotherapeutic concepts propose to foster the biosynthesis of beneficial SPMs. Here, we demonstrate that the natural combination medicine Traumeel (Tr14) improves resolution of inflammation by promoting SPM formation. Tr14 enhanced the biosynthesis of 12-/15-lipoxygenase (LOX) products and of SPMs in zymosan-induced mouse peritonitis as well as in human monocyte-derived macrophages challenged with Staphylococcus aureus. Importantly, in the peritonitis model, Tr14 supported the recruitment of innate leukocytes and the efferocytotic capacity of macrophages, and positively influenced the inflammation resolution index. Taken together, we suggest that based on these properties Tr14 may possess therapeutic potential as an enhancer for the resolution of inflammatory processes.
Keywords: Traumeel; efferocytosis; inflammation; inflammation resolution; lipid mediator; multicomponent; peritonitis; resolution index; specialized pro-resolving mediators.
1. Introduction
2. Results
2.1. Tr14 Affects Lipid Mediator Pathways and Promotes Resolution of Inflammation in Murine Peritonitis
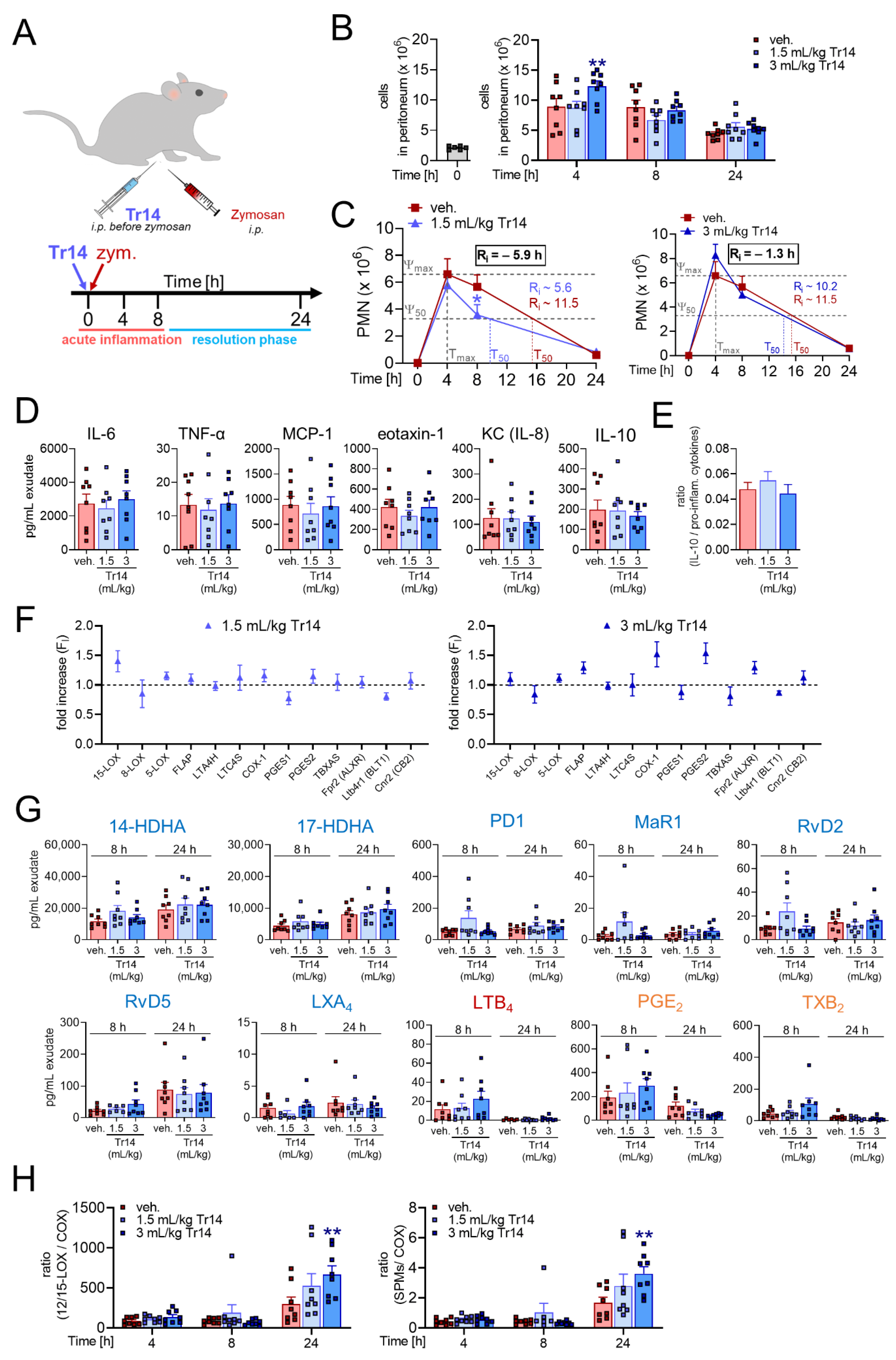
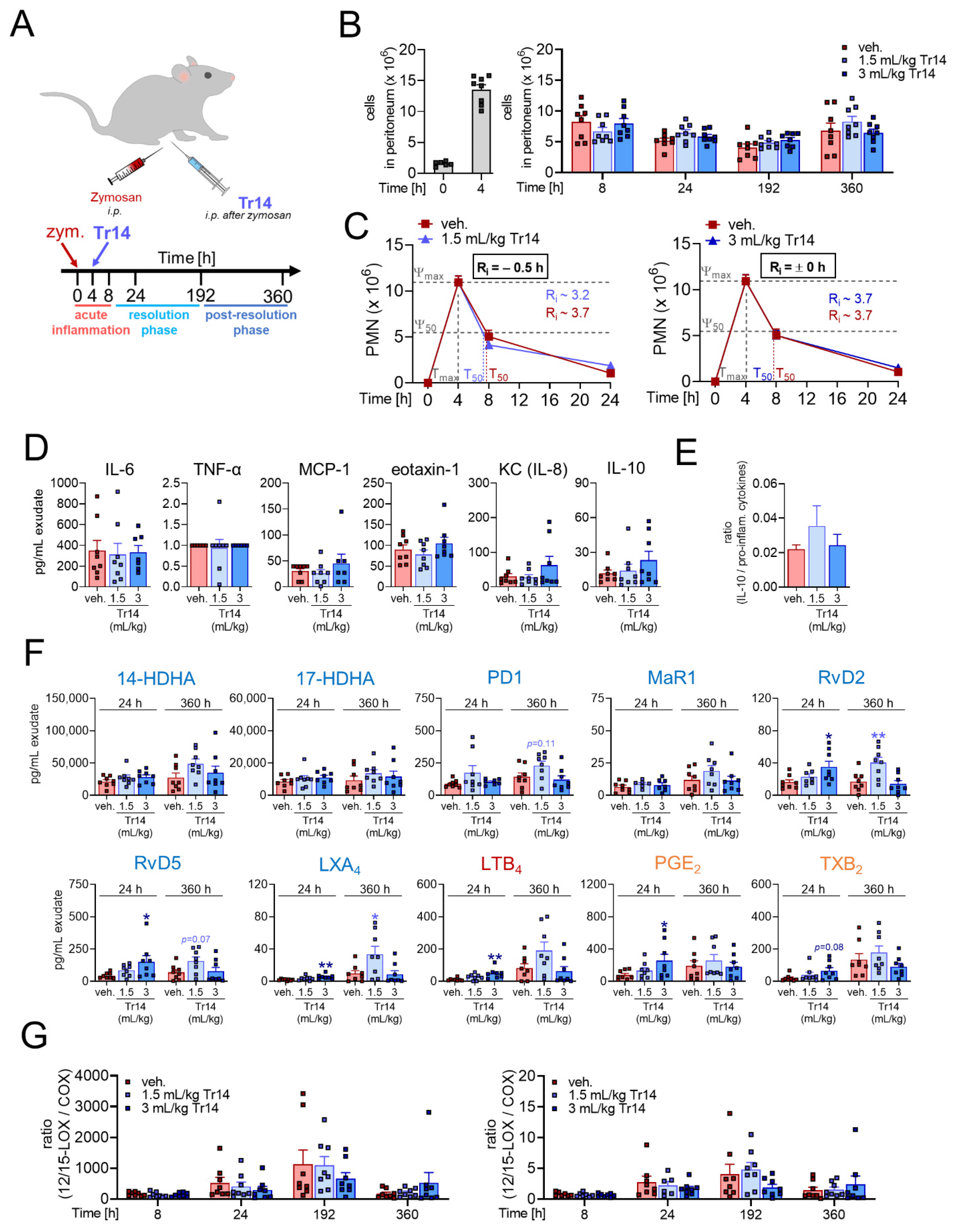
2.2. Tr14 Enhances Macrophage Efferocytotic Actions In Vivo

2.3. Tr14 Increases 15-Lox-1-Mediated Lipid Mediator Biosynthesis in Human M2 Macrophages
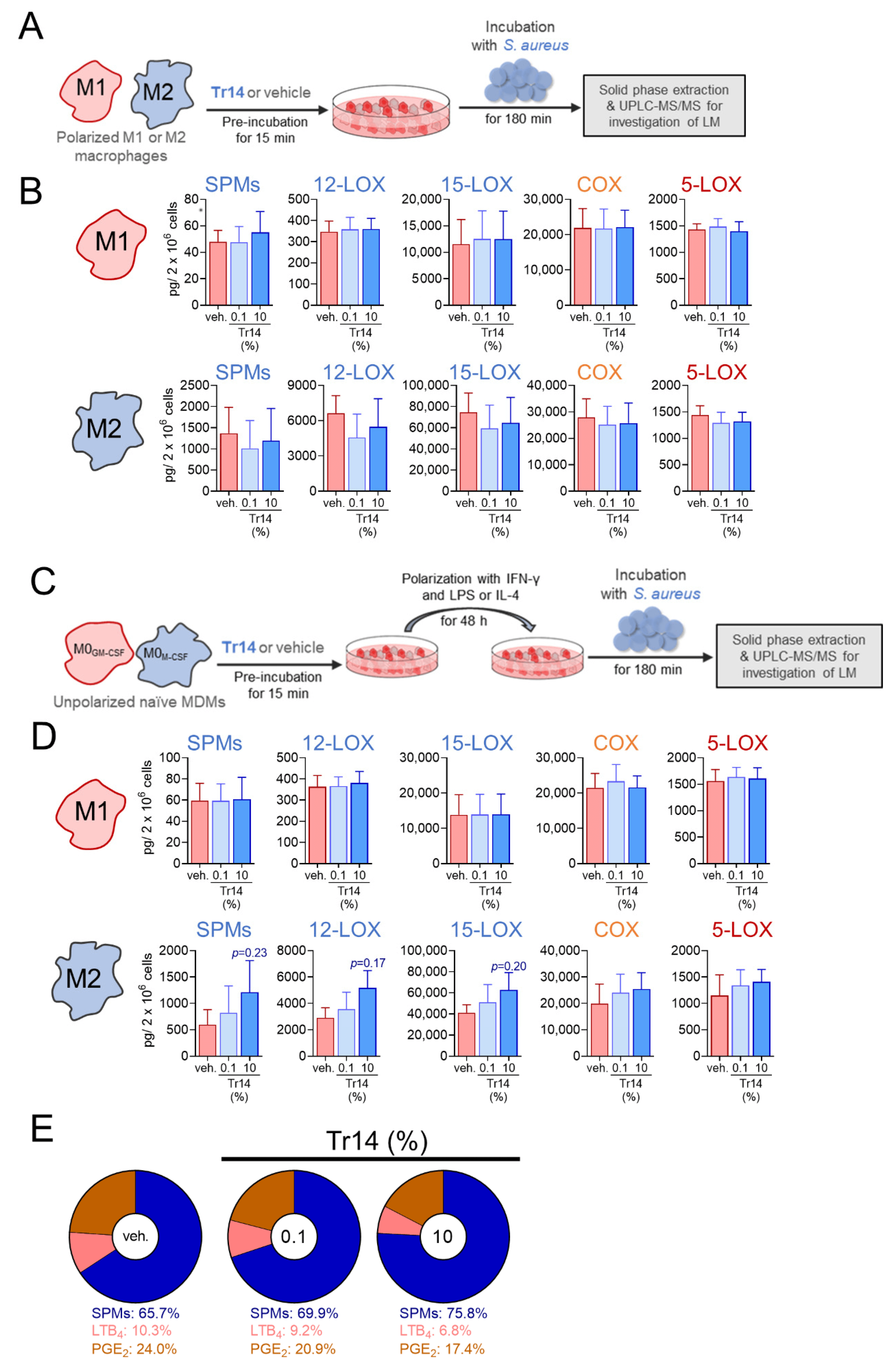
3. Discussion
4. Materials and Methods
4.1. Interventions
4.2. Animals, Animal Care and the Ethical Statement
4.3. Study Design
4.4. Zymosan-Induced Murine Peritonitis and Peritoneal Lavage Collection
4.5. Flow Cytometry and Definition of the Resolution Index
4.6. Measurement of Lipid Mediators and Cytokines in Peritoneal Lavage
4.7. Measurement of Gene Expression in the Peritoneal Lavage
4.8. Isolation of Human Monocytes and Preparation of Monocyte-Derived Macrophages
4.9. Bacterial Cultivation
4.10. Cytotoxicity Analysis
4.11. Incubation of Human MDM and LM Metabololipidomics
4.12. Quantification and Statistical Analysis
5. Conclusions
Supplementary Materials
Author Contributions
Funding
Institutional Review Board Statement
Informed Consent Statement
Data Availability Statement
Acknowledgments
Conflicts of Interest
References
- Rainsford, K. Anti-Inflammatory Drugs in the 21st Century. Subcell. Biochem. 2007, 42, 3–27. [Google Scholar] [CrossRef]
- Patrignani, P.; Patrono, C. Cyclooxygenase inhibitors: From pharmacology to clinical read-outs. Biochim. Biophys. Acta-Mol. Cell Biol. Lipids 2015, 1851, 422–432. [Google Scholar] [CrossRef]
- Gilroy, D.; Colvillenash, P.R.; Willis, D.K.; Chivers, J.; Paulclark, M.J.; Willoughby, D. Inducible cyclooxygenase may have anti-inflammatory properties. Nat. Med. 1999, 5, 698–701. [Google Scholar] [CrossRef]
- Nathan, C. Points of control in inflammation. Nature 2002, 420, 846–852. [Google Scholar] [CrossRef]
- Lawrence, T.; Willoughby, D.A.; Gilroy, D. Anti-inflammatory lipid mediators and insights into the resolution of inflammation. Nat. Rev. Immunol. 2002, 2, 787–795. [Google Scholar] [CrossRef]
- Serhan, C.N.; Savill, J. Resolution of inflammation: The beginning programs the end. Nat. Immunol. 2005, 6, 1191–1197. [Google Scholar] [CrossRef]
- Serhan, C.N.; Brain, S.; Buckley, C.D.; Gilroy, D.; Haslett, C.; O’Neill, L.; Perretti, M.; Rossi, A.G.; Wallace, J.L. Resolution of in flammation: State of the art, definitions and terms. FASEB J. 2007, 21, 325–332. [Google Scholar] [CrossRef] [Green Version]
- Chiang, N.; Fredman, G.; Bäckhed, F.; Oh, S.F.; Vickery, T.; Schmidt, B.A.; Serhan, C.N. Infection regulates pro-resolving mediators that lower antibiotic requirements. Nat. Cell Biol. 2012, 484, 524–528. [Google Scholar] [CrossRef] [Green Version]
- Newson, J.; Stables, M.; Karra, E.; Arce-Vargas, F.; Quezada, S.; Motwani, M.; Mack, M.; Yona, S.; Audzevich, T.; Gilroy, D.W. Resolution of acute inflammation bridges the gap between innate and adaptive immunity. Blood 2014, 124, 1748–1764. [Google Scholar] [CrossRef] [Green Version]
- Buckley, C.D.; Gilroy, D.; Serhan, C.N. Proresolving Lipid Mediators and Mechanisms in the Resolution of Acute Inflammation. Immunity 2014, 40, 315–327. [Google Scholar] [CrossRef] [Green Version]
- Gao, L.; Faibish, D.; Fredman, G.; Herrera, B.S.; Chiang, N.; Serhan, C.N.; Van Dyke, T.E.; Gyurko, R. Resolvin E1 and Chemokine-like Receptor 1 Mediate Bone Preservation. J. Immunol. 2013, 190, 689–694. [Google Scholar] [CrossRef] [PubMed] [Green Version]
- Serhan, C.N. Pro-resolving lipid mediators are leads for resolution physiology. Nature 2014, 510, 92–101. [Google Scholar] [CrossRef] [PubMed] [Green Version]
- Funk, C.D. Prostaglandins and Leukotrienes: Advances in Eicosanoid Biology. Science 2001, 294, 1871–1875. [Google Scholar] [CrossRef] [PubMed] [Green Version]
- Bennett, M.; Gilroy, D. Lipid Mediators in Inflammation. Microbiol. Spectr. 2016, 4. [Google Scholar] [CrossRef]
- Bannenberg, G.; Chiang, N.; Ariel, A.; Arita, M.; Tjonahen, E.; Gotlinger, K.H.; Hong, S.; Serhan, C.N. Molecular Circuits of Resolution: Formation and Actions of Resolvins and Protectins. J. Immunol. 2005, 174, 4345–4355. [Google Scholar] [CrossRef] [Green Version]
- Schwab, J.; Chiang, N.; Arita, M.; Serhan, C.N. Resolvin E1 and protectin D1 activate inflammation-resolution programmes. Nat. Cell Biol. 2007, 447, 869–874. [Google Scholar] [CrossRef] [Green Version]
- Dinarello, C.A. Anti-inflammatory Agents: Present and Future. Cell 2010, 140, 935–950. [Google Scholar] [CrossRef] [Green Version]
- Serhan, C.N.; Dalli, J.; Colas, R.A.; Winkler, J.W.; Chiang, N. Protectins and maresins: New pro-resolving families of mediators in acute inflammation and resolution bioactive metabolome. Biochim. Biophys. Acta-Mol. Cell Biol. Lipids 2015, 1851, 397–413. [Google Scholar] [CrossRef] [Green Version]
- Lussignoli, S.; Bertani, S.; Metelmann, H.; Bellavite, P.; Conforti, A. Effect of Traumeel S®, a homeopathic formulation, on blood-induced inflammation in rats. Complement. Ther. Med. 1999, 7, 225–230. [Google Scholar] [CrossRef]
- Laurent, G.I.S.; Seilheimer, B.; Tackett, M.; Zhou, J.; Shtokalo, D.; Vyatkin, Y.; Ri, M.; Toma, I.; Jones, D.; McCaffrey, T.A. Deep Sequencing Transcriptome Analysis of Murine Wound Healing: Effects of a Multicomponent, Multitarget Natural Product Therapy-Tr14. Front. Mol. Biosci. 2017, 4, 57. [Google Scholar] [CrossRef] [PubMed]
- Žilinskas, J.; Žekonis, J.; Žekonis, G.; Šadzevičienė, R.; Sapragonienė, M.; Navickaitė, J.; Barzdžiukaitė, I. Inhibition of peripheral blood neutrophil oxidative burst in periodontitis patients with a homeopathic medication Traumeel S. Med. Sci. Monit. 2011, 17, CR284–CR291. [Google Scholar] [CrossRef] [PubMed] [Green Version]
- Baldwin, A.L.; Bell, I.R. Effect of noise on microvascular integrity in laboratory rats. J. Am. Assoc. Lab. Anim. Sci. 2007, 46, 58–65. [Google Scholar]
- Muders, K.; Pilat, C.; Deuster, V.; Frech, T.; Krüger, K.; Pons-Kühnemann, J.; Mooren, F.-C. Effects of Traumeel (Tr14) on recovery and inflammatory immune response after repeated bouts of exercise: A double-blind RCT. Graefe’s Arch. Clin. Exp. Ophthalmol. 2017, 117, 591–605. [Google Scholar] [CrossRef]
- Pilat, C.; Frech, T.; Wägner, A.; Krüger, K.; Hillebrecht, A.; Pons-Kühnemann, J.; Scheibelhut, C.; Bödeker, R.-H.; Mooren, F.-C. Exploring effects of a natural combination medicine on exercise-induced inflammatory immune response: A double-blind RCT. Scand. J. Med. Sci. Sports 2014, 25, 534–542. [Google Scholar] [CrossRef] [PubMed]
- Le Faouder, P.; Baillif, V.; Spreadbury, I.; Motta, J.-P.; Rousset, P.; Chêne, G.; Guigné, C.; Tercé, F.; Vanner, S.; Vergnolle, N.; et al. LC–MS/MS method for rapid and concomitant quantification of pro-inflammatory and pro-resolving polyunsaturated fatty acid metabolites. J. Chromatogr. B 2013, 932, 123–133. [Google Scholar] [CrossRef]
- Serhan, C.N.; Chiang, N.; Van Dyke, T.E. Resolving inflammation: Dual anti-inflammatory and pro-resolution lipid mediators. Nat. Rev. Immunol. 2008, 8, 349–361. [Google Scholar] [CrossRef] [Green Version]
- Werz, O.; Gerstmeier, J.; Libreros, S.; De La Rosa, X.; Werner, M.; Norris, P.; Chiang, N.; Serhan, C.N. Human macrophages differentially produce specific resolvin or leukotriene signals that depend on bacterial pathogenicity. Nat. Commun. 2018, 9, 59. [Google Scholar] [CrossRef]
- Werner, M.; Jordan, P.M.; Romp, E.; Czapka, A.; Rao, Z.; Kretzer, C.; Koeberle, A.; Garscha, U.; Pace, S.; Claesson, H.E.; et al. Targeting biosynthetic networks of the proinflammatory and proresolving lipid metabolome. FASEB J. 2019, 33, 6140–6153. [Google Scholar] [CrossRef]
- Ghlichloo, I.; Gerriets, V. Nonsteroidal Anti-Inflammatory Drugs (NSAIDs); StatPearls Publishing LLC.: Treasure Island, FL, USA, 2021. [Google Scholar]
- Sehajpal, S.; Prasad, D.N.; Singh, R. Prodrugs of Non-steroidal Anti-inflammatory Drugs (NSAIDs): A Long March towards Synthesis of Safer NSAIDs. Mini Rev. Med. Chem. 2018, 18, 1199–1219. [Google Scholar] [CrossRef] [PubMed]
- Serhan, C.N.; Levy, B.D. Resolvins in inflammation: Emergence of the pro-resolving superfamily of mediators. J. Clin. Investig. 2018, 128, 2657–2669. [Google Scholar] [CrossRef]
- Dalli, J.; Serhan, C. Macrophage Proresolving Mediators—The When and Where. Microbiol. Spectr. 2016, 4, 367–383. [Google Scholar] [CrossRef] [PubMed] [Green Version]
- Dalli, J.; Serhan, C.N. Pro-Resolving Mediators in Regulating and Conferring Macrophage Function. Front. Immunol. 2017, 8, 1400. [Google Scholar] [CrossRef] [Green Version]
- Jordan, P.M.; Gerstmeier, J.; Pace, S.; Bilancia, R.; Rao, Z.; Börner, F.; Miek, L.; Gutiérrez-Gutiérrez, Ó.; Arakandy, V.; Rossi, A.; et al. Staphylococcus aureus-Derived α-Hemolysin Evokes Generation of Specialized Pro-resolving Mediators Promoting Inflammation Resolution. Cell Rep. 2020, 33, 108247. [Google Scholar] [CrossRef] [PubMed]
- Ji, R.-R.; Xu, Z.-Z.; Strichartz, G.; Serhan, C.N. Emerging roles of resolvins in the resolution of inflammation and pain. Trends Neurosci. 2011, 34, 599–609. [Google Scholar] [CrossRef] [Green Version]
- Lozada, C.J.; Del Rio, E.; Reitberg, D.P.; Smith, R.A.; Kahn, C.B.; Moskowitz, R.W. A double-blind, randomized, saline-controlled study of the efficacy and safety of co-administered intra-articular injections of Tr14 and Ze14 for treatment of painful osteoarthritis of the knee: The MOZArT trial. Eur. J. Integr. Med. 2017, 13, 54–63. [Google Scholar] [CrossRef]
- Miller, R.; Belmadani, A.; Ishihara, S.; Tran, P.B.; Ren, D.; Miller, R.J.; Malfait, A.-M. Damage-Associated Molecular Patterns Generated in Osteoarthritis Directly Excite Murine Nociceptive Neurons Through Toll-like Receptor 4. Arthritis Rheumatol. 2015, 67, 2933–2943. [Google Scholar] [CrossRef]
- De Vega, C.G.; Speed, C.; Wolfarth, B.; González, J. Traumeel vs. diclofenac for reducing pain and improving ankle mobility after acute ankle sprain: A multicentre, randomised, blinded, controlled and non-inferiority trial. Int. J. Clin. Pract. 2013, 67, 979–989. [Google Scholar] [CrossRef] [Green Version]
- Colas, R.A.; Shinohara, M.; Dalli, J.; Chiang, N.; Serhan, C.N. Identification and signature profiles for pro-resolving and inflammatory lipid mediators in human tissue. Am. J. Physiol. Physiol. 2014, 307, C39–C54. [Google Scholar] [CrossRef] [PubMed] [Green Version]